Autumn is here, which means summer colors are beginning to fade and many leaves are starting to change from green to red and yellow. Although it’s all around us, we might not often think about what color actually is. Color is the characteristic that describes the light waves that are absorbed by, and bounce off, an object. 1 There are two ways color can occur: either pigment, like the indigo dye used to color blue jeans, or nanostructures, like in butterfly wings!
You might not realize it, but these colors are telling us something about chemistry and biology. For example, green returning to the leaves in spring indicates the health of the plant and the presence of the green pigment chlorophyll. The change in color in leaves during autumn is the result of the breakdown of the chlorophyll, revealing the other beautiful pigments in the leaves.2 Perhaps the most dazzling display of color comes from the biological fireworks of flowers. Flowers can come in a whole range of colors in the visible spectrum (and in fact have special patterns only revealed by ultraviolet light!).

Flowers’ many colors come from a variety of natural chemical pigments that reflect or absorb certain colors of light, causing us to see particular colors. Some flowers can even change colors! Hydrangeas, for example, can change between pink and blue because of special kinds of natural chemical pigments, called anthocyanins. Not only can chemistry help explain why we see different colors in nature, but chemists can also use color to answer a ton of different chemical and biological questions!

Regardless of whether color is coming from pigments (like paints in a picture or dye in textiles) or nanostructures (like butterfly wings or nano-gold), what the receptors in our eyes are registering are certain wavelengths of light that are not absorbed, but reflected, by the pigments or structures of the material. Since color is something perceived, the quality of color might seem debatable considering that two different people might describe a single color in different ways. But, because the color comes from light with specific wavelengths, there are ways to measure color! You might have experience describing a color using the name of a Crayola crayon, or with the RGB values on a computer, but colors can also be precisely reported as a single wavelength measured in nanometers.
In a laboratory, scientists use special instruments to help determine the precise nanometer wavelengths of a color. One of those instruments is a spectrophotometer.

Spectrophotometers measure the light that is absorbed or transmitted by a material, which can tell us a lot about the material. Instruments like this can even be used to measure the distance of stars!

Scientists use these instruments not only to determine the identity of a color, but to watch a color develop over time or measure how intense it is. The power of color analysis can be harnessed to help give answers to any number of scientific questions: How much protein is in here? How far has this reaction gone? How big are these nanoparticles? Although we may not use spectrophotometers in our everyday lives, we do use color changes to indicate chemical properties at home. For example, if you cut an apple, potato, or avocado and notice it turns brown, that’s an example of a chemical reaction, called oxidation, that leads to color change. Cooking egg whites is another example: as they change from clear to white, you know that your egg is cooked!

Acids and Bases
When thinking about how color can inform us about chemistry, perhaps the most typical example is assessing pH. pH is the measure of how acidic or basic something is. There are several different ways to define what an acid or base is, but we’ll stick with one definition for ease: the Arrhenius definition. Nineteenth Century chemist Svante Arrhenius defined acids as substances that dissociate (break apart) and generate H+ or H3O+ (a proton or hydronium ion) in water, while a base would dissociate and generate OH– (a hydroxide ion). The measurement of pH is based on how much H+ or OH– is generated, with acids being closer to 1, bases closer to 14, and something neutral (like water!) in between at 7. pH can affect surrounding molecules and other chemical reactions. For example, remember our oxidation example from apples and avocados? Adding acids like lemon juice on a cut apple, or lime on an avocado, can help prevent the browning effect.
Because of the influence pH can have, it is important to measure and keep it constant in the research lab so that we don’t add an extra variable to whatever we’re studying. For example, in the Center for Sustainable Nanotechnology, it is important to keep pH controlled when we analyze nanomaterials or study organisms. pH can affect nanoparticle properties such as how they dissolve, which also determines the effect they will have on organisms like bacteria. Even plants are sensitive to pH changes in the soil (like our hydrangea example), so it’s also important to control pH when we’re studying how nanoparticles interact with plants.
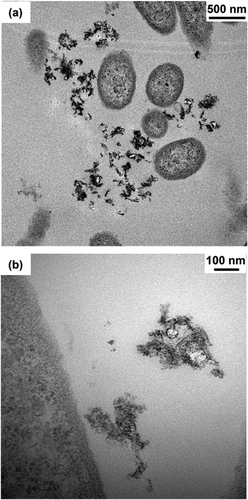
How do we measure pH?
We can measure pH in the lab in a couple ways:
- Use a pH meter, a device that measures the electric potential between two electrodes in order to quantify the amount of H+ in a solution. This is the most precise and accurate way to measure pH.
- Use a pH indicator, which is the “quick and dirty” way to get a general idea of the pH of a solution. pH indicators can come in both liquid and paper form, so you can either drop some indicator in your liquid of interest and look for a color change, or you can dip color changing paper in your liquid of interest and look for the paper to change color. (You may have used these paper pH indicators if you have a fish tank.

One of the first examples of pH paper is Litmus paper. You may have heard the word litmus before, likely in reference to a litmus test, colloquially meaning a way to test someone’s beliefs – this comes from chemistry! Litmus is actually a blue dye that is extracted from lichens, which are a community of algae or cyanobacteria living with fungi that you might see commonly living on trees or rocks. In fact, the word litmus comes from the Old Norse Word litmose (lita = to dye or stain ; mos = moss)

Litmus was originally made from a combination of multiple lichens but is now mostly made from two main species, and still contains numerous different dyes. To get the dye from the lichens, the lichens are collected and ground with sodium carbonate (Na2CO3, commonly known as “washing salt”) and ammonia (NH3, a solvent to dissolve the lichens and salt). After several weeks, the solution is dried, and the litmus is separated from other dyes with an alcohol extraction. The litmus can then be used as a liquid indicator or absorbed into paper to generate litmus paper. pH indicators like litmus change color based on the pH of the liquid they’re in contact with. Litmus is red under acidic conditions and blue under alkaline, being able to change colors based on pH in the range of 4.5-8.3.5,6
But lichens aren’t the only natural items that can be used to make a pH indicator. There are even things in your house that can be used to indicate pH! Red cabbage is perhaps the most well-known at-home indicator. Similar to lichens, the color component in red cabbage can be extracted, is pH sensitive, and can dye paper. And there are plenty of items in your home that are acidic or basic so you can test your at-home indicators. You can use ingredients like baking soda (a base) and vinegar (an acid) to change the pH – and thereby the color – of your cabbage extract. Low pH (acid) appears red with cabbage indicator, more neutral pH looks purple, and high pH (base) will turn blue and eventually green.
The chemicals responsible for these pH-dependent color changes are called anthocyanins, the same kinds pigments found in hydrangea flowers. There are multiple different kinds of anthocyanins, and they are responsible for a lot of the red, purple, or blue colors of different plants. The anthocyanin responsible for the color change in red cabbage is called cyanidin.7 Other purple, red, or blue plants may have different anthocyanins or even multiple. Blueberries have at least 4 kinds different anthocyanins.8

The bright colors in plants are from chemicals that occur naturally. Of course, there are synthetic compounds that humans can use to artificially color fruits and vegetables to make them more appealing, but generally, the colors in fruits and vegetables come from naturally occurring chemicals. If you’re interested in experimenting with cabbage and other produce with natural pigments, check out the activity on our new Nano at Home page!
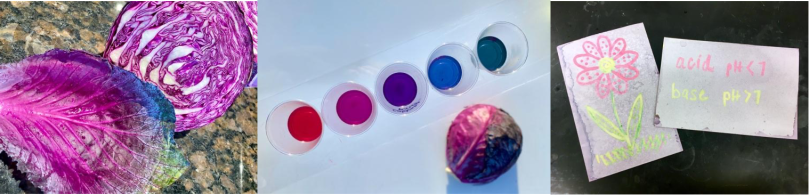
Educational Resources
- Apple browning experiment
- Lifeology course about the importance of eating fruits and veggies
- Extracting pigments from fall leaves
- Exploratorium: Truly Primary Pigments activity
- Classroom activity for plant pigment identification
REFERENCES
- How Stuff Works. How Light Works. https://science.howstuffworks.com/light12.htm
- SciJinks. Why Do Leaves Change Color? https://scijinks.gov/leaves-color/
- Schulte, A.J. et al. Ultraviolet patterns of flowers revealed in polymer replica – caused by surface architecture. Nanotechnology 2019, 10, 459–466. doi: 10.3762/bjnano.10.45
- Hang, M.* & Gunsolus, I.* et al. Impact of Nanoscale Lithium Nickel Manganese Cobalt Oxide (NMC) on the Bacterium Shewanella oneidensis MR-1. Chemistry of Materials, 2016, 28 (4), 1092-1100. doi: 10.1021/acs.chemmater.5b04505 (* these authors contributed equally).
- ThoughtCo. Litmus Paper and the Litmus Test. https://www.thoughtco.com/what-is-litmus-paper-3976018
- How Products are Made. Litmus Paper. http://www.madehow.com/Volume-6/Litmus-Paper.html
- Wiczkowski, W. et al. Changes in the content and composition of anthocyanins in red cabbage and its antioxidant capacity during fermentation, storage and stewing. Food Chemistry, 2015, 167, 115-123. doi: 10.1016/j.foodchem.2014.06.087
- Lohachoompol, V. et al. Anthocyanins—More Than Nature’s Colour. Journal of Biomedical Biotechnology, 2004, 2004, 248-252. doi: 10.1155/S1110724304406123