You’ve read a lot on this blog about how nanoparticles provide amazing opportunities for developing new materials. Over the next few years, we will have incredible advances in sustainable energy, biology, and more based on these new substances that never existed before. But what about the old, structural materials used in our buildings and cars? Well, nano is going to play a big role in the sustainability of those too!
First, a bit of a background on what makes materials good for these applications: that is, what makes them strong and stand the test of time? For metals, their strength comes from the way their atoms fit together. They’re held together by a strong “metallic bond”. Unlike the other types of bonds, in metallic bonds all of the atoms share their electrons with each other. This allows for some of the properties you probably identify with metals – conduction of heat and electricity. The bonds also lack any directionality requirements, and this lets the atoms fit together in very space efficient ways like you could see in a grocery store aisle’s oranges or the bubbles that form on the top of some sudsy water. Space efficiency in this case means that the objects fit together in a way that minimizes the empty space around each of them.

Because of the strong bonds and the close arrangement of the atoms in metals, they really effectively resist being moved around. So, in our day-to-day lives, we see this as metals being “strong” or resistant to damage. This is one of the big reasons we use metals in major load-bearing applications like buildings and cars. We can’t have those atoms moving around and making the whole structure change its shape under pressure!
The other main factor we need to think about in these applications is material’s corrosion resistance. Corrosion comes from materials chemically reacting with their environment. The most commonly recognized form of this is rust – the red, scaly stuff you might see on old cars or nails left outside too long. Rust is an oxide formed when iron reacts with oxygen in air and water. As you probably know from your own experiences with it, it’s not a good thing to have happen to something you want to stay strong. The rust easily flakes off and leaves less metal behind than before the rust was made. That means using iron may not be the best choice for applications where there might be a lot of interaction with air and water!

So, if you want a sustainable structure – one that you don’t have to rebuild every few years because it’s falling apart from corrosion – you really want to be sure the metal can resist corrosion. One of the best ways this is done already is by adding in other elements to the mix that create a different material instead of rust when it reacts with air and water. For instance, chromium is usually added to iron along with other elements to make stainless steel. The chromium reacts more readily than any of the other elements to make its oxide, and instead of flaking off, this oxide keeps air and water out right after it’s made. So, essentially it creates its own shield from further corrosion. That means only a very small layer is converted from the original metal, and then it stays the same from then on. This is called “passivation”. This is also why aluminum is used for a lot of applications – it makes one of these shielding layers on its own, without having to mix in any other material. Unfortunately, both stainless steel and aluminum have their own issues: steel is pretty heavy for all its well-known strength, and aluminum isn’t nearly as strong as steel so it can’t be used for as many load-bearing applications like the frames of buildings. Having to select for corrosion resistance definitely limits our options for building materials!
You may be wondering where nano comes into this. Yes, there have been many advancements in metals already that allow for a lot of applications. But they have limits! Like aluminum, some just aren’t strong enough for the weight we want to put on them, and others continue to corrode in particular environments even after forming protective layers. Nanocrystallinity has the potential to solve both of these problems.
Most metals have sub structures within them called grains or “crystallites”. These are regions within which atoms are ordered efficiently (like the oranges and bubbles shown above). However, when a metal is made, it doesn’t always have every single atom aligned together uniformly throughout in what is considered a single crystal. In fact, this is really hard to make happen! So most metals have regions with different ordering that all together make up the total structure. These metals are called polycrystalline because they are made of multiple grains or crystallites with different orientations.1

These grains aren’t necessarily a problem – it turns out that having lots of grains often actually improves both the strength and the corrosion resistance of metals. The boundaries between the grains keep any deformation from propagating through the material (it gets held up at the grain borders) and the grain edges are also areas of increased rates of production for protective passivating layers.2 It makes sense, then, that more grain boundaries is better for both strength and corrosion resistance.
So how do you get more grain boundaries per volume of material? You shrink the grains! The name used for the strengthening effect from decreasing grain size (and thus increasing grain boundaries) is Hall-Petch strengthening,3 and it can work all the way up until grains are only nanometers in size – nanocrystalline. To give an idea of the scale of these materials, in 1 cubic centimeter of a nanocrystalline metal, there can be as many as a quadrillion (10^15) grains! Compare this to typical metals which can have anywhere from a few million (10^6) to even just a handful of grains in 1 cubic centimeter.
Similarly, studies have recently shown a (not yet named) relationship where decreasing the grain size also increases the resistance to corrosion.4 Many researchers are showing every day that nanocrystalline metals have extremely high strength5 and very high resistance to corrosion.6
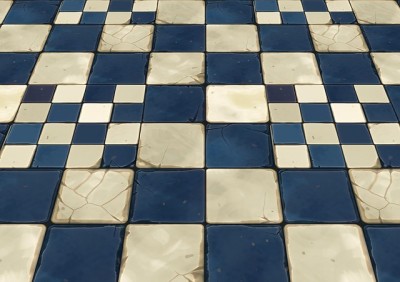
There are still some issues with nanocrystalline metals. The small grain sizes are not always very stable, and, similar to some nanoparticles, the grains like to group together and grow into bigger grains instead of staying small. When this occurs, the beneficial properties decrease. Also, with the increased strength of these materials, they often lose some of the ability to bend under pressure instead of breaking that metals are known to have. This can be a problem for some applications; for instance, we prefer our cars to crumple in an accident instead of breaking since the crumpling absorbs the energy of the collision and keeps the passengers safe. So we might not use nanocrystalline metals in car frames.
Nanocrystalline metals are already starting to see use in coatings that keep the underlying material safe from damage and corrosion.7 In the long-term, because of their increased strength and corrosion resistance, these coated items will need to be replaced less and last longer than items made with traditional metals. Once the issues with ductility and stability have been worked out for some systems, they will likely find even more use in a wide array of structural applications. A hundred years from now, we may no longer need to make and buy new cars and buildings because they will have been built to last with nanocrystalline metals!
This week’s post is by guest blogger Alex Bryant. Alex is a Ph.D. student at Georgia Tech in the School of Materials Science and Engineering. When not researching the structural and mechanical responses of exotic metals to high-powered lasers, he likes to watch TV, read books, and backpack in the mountains of North Georgia.
REFERENCES (some may need subscriptions for full access)
1. Wikipedia. Crystal. http://en.wikipedia.org/wiki/Crystal (accessed April 7, 2015).
2. Lee, S.; White, H. S. Dissolution of the Native Oxide Film on Polycrystalline and Single-Crystal Aluminum in NaCl Solutions. J. Electrochem. Soc. 2004, (8) B479-B483. DOI: 10.1149/1.1766309
3. Wikipedia. Grain Boundary Strengthening. http://en.wikipedia.org/wiki/Grain_boundary_strengthening (accessed April 7, 2015).
4. Ralston, K. D.; Birbilis, N.; Davies, C. H. J. Reveailing the relationship between grain size and corrosion rate of metals. Scripta Mat. 2010, 63, 1201-1204. DOI: 10.1016/j.scriptamat.2010.08.035
5. Kumar, K. S.; Swygenhoven, H. V.; Suresh, S. Mechanical behavior of nanocrystalline metals and alloys. Acta Materialia 2003, 51, 5743-5774. DOI: 10.1016/j.actamat.2003.08.032
6. Gu, C.; Lian, J.; He, J.; Jiang, Z.; Jiang, Q. High corrosion-resistance nanocrystalline Ni coating on AZ91D magnesium alloy. Surface and Coatings Technology 2006, 200, 5413-5418. DOI: 10.1016/j.surfcoat.2005.07.001
7. Schuh, C.; Lund, A.. Methods for the Implementation of Nanocrystalline and Amorphous Metals and Alloys as Coatings. (Xtalic Corporation, Marlborough, MA). Patent 7521128. N.d. Print.